Climate Insight Series
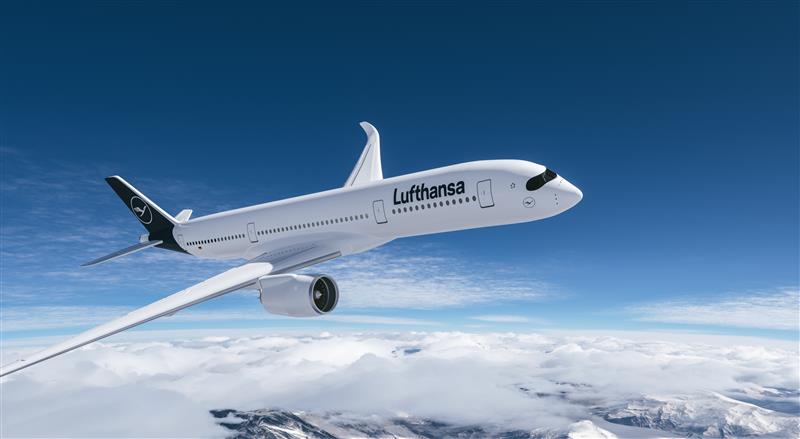
Aviation stands at a critical crossroads: while connecting economies and cultures by transporting around 9 billion passengers annually1, the sector currently contributes 2-3% of global greenhouse gas emissions (GHGs)2. Unlike other industries making rapid progress toward decarbonisation, aviation’s emissions are projected to grow significantly without intervention. This diverging pathway creates both urgent challenges and unprecedented opportunities for industry transformation. In this article, we outline the technological, operational and policy pathways to reducing GHG emissions.
Figure 1: Global CO₂ emissions from transport by sub-sector in the Net-Zero Scenario, 2000-2030
Source: IEA (2023), Global CO₂ emissions from transport by sub-sector in the Net-Zero Scenario, 2000-2030, IEA, Paris https://www.iea.org/data-and-statistics/charts/global-CO₂-emissions-from-transport-by-sub-sector-in-the-net-zero-scenario-2000-2030-2, Licence: CC BY 4.0
What are the issues?
Aviation's carbon footprint primarily comes from the burning of fossil fuels during aircraft operations. Carbon dioxide (CO₂) is the major greenhouse gas emitted by aircraft engines, along with other emissions contributing to the industry's environmental impact, such as nitrous oxide (N₂O). The fact that the emissions are generated high in the atmosphere is also thought to affect climate and potentially add to the sector’s climate impact.
While passenger demand for air travel is expected to grow at around 3% annually (with lower growth in Europe and higher growth in Asia Pacific),3 technological innovation cycles in aviation typically span 15-20 years – creating a critical timing challenge for decarbonisation efforts. According to the scenarios modelled by the International Council on Clean Transportation in its Vision 2050: Aligning Aviation with the Paris Agreement report, for aviation to align with the Paris Agreement of limiting the global temperature increase to 2°C and ideally 1.5°C above pre-industrial levels, the sector’s cumulative CO₂ emissions budget from 2020 to 2050 is 33.8Gt and 11.5Gt respectively4 but from 2020 to 2023, the sector’s cumulative emissions were 3Gt, eating into a substantial portion of its remaining 1.5°C aligned carbon budget. To align with 2°C and 1.5°C scenarios, the sector would need to reduce emissions by 30% and 76% by 2050 with a 2020 baseline, and residual emissions must be offset elsewhere in the economy.
Figure 2: Cumulative emissions under ICCT global aviation scenarios from 2020 to 2050
Source: ICCT Aviation 2050 Report
What’s the solution?
Utilisation of sustainable aviation fuel (SAF), introduction of zero emission planes (ZEP), and increase of operational and technical efficiency are all effective measures for corporate’s net-zero goals. SAF is expected to be the key contributor to the sector’s decarbonisation pathway, followed by ZEP, and technical and operational efficiency. Currently, improving technical and operational efficiency is a short-term solution that has reached its natural limit, and SAF is considered the solution for the medium-term before zero emission planes can fully scale up.
Technology |
Transition Readiness Level |
Mitigation potential |
Cost5 |
SAF |
Commercial operation in relevant environment |
High |
High |
Zero emissions planes |
Full prototype at scale |
High |
High |
Operational & technical efficiencies |
Market Ready |
Moderate |
Low |
Key Decarbonisation Pathways
Sustainable Aviation Fuel
In the short to medium-term, SAF will likely play a key decarbonisation role. There are two main sustainable aviation fuel types: biofuels and synthetic fuels. Biofuels are produced from sustainable feedstocks, such as corn grain and oil seeds, used cooking oil, agricultural residues, and household waste, while synthetic fuels are produced through combining captured carbon and green hydrogen. SAFs currently achieve an average 70% lifecycle CO₂ reduction compared to conventional jet fuel.6 It is blended with conventional kerosene without modification to aircraft, with a 10 to 50% blending limitation to meet the ASTM International Standards.7 Biofuels are more favorable as a solution in the short-term compared to synthetic fuels. Algae is considered the most ideal feedstock to produce large quantities of SAF, given it absorbs carbon dioxide to grow and produces up to 15 times more oil per square kilometer than other biofuel crops.8
The engine manufacturers are working on enabling 100% capacity of SAF intake for future. To achieve the net-zero goal by 2050, annual supply of SAF needs to reach 450 billion liters—equivalent to 1.5 billion tons of algae biomass—while current supply is only 0.07% of global jet fuel demand.9,10
Challenges to production scale include the complexity in supply chain and the need for technology and infrastructure improvement. SAFs have to compete with other industries such as food production and chemicals for the availability of sustainable feedstock resources, and the cost of feedstock can vary significantly, which impacts the economic viability of SAF production. Enhancing production methods and modifying current or building new refineries are also important to scale up SAF production volume. In addition, using renewable energy is essential to ensure the environmental benefits of SAF.
Zero emission planes (hydrogen + electric)
Zero emission planes are the holy grail for the sector. Today, examples of both battery-electric and hydrogen fuel cell planes exist but their commercial application lags far behind other transport sectors. Though battery densities and range potential are continually increasing over the long-term, it is expected to only be an option for short-haul flights. Another developing low-carbon technology for short-haul flights is hydrogen fuel cell planes.
Hydrogen provides three times the energy per unit mass compared to fossil fuel and produces zero carbon emissions, but hydrogen-powered aircraft currently lasts approximately half of gas-fueled plane’s range. This is due to hydrogen’s low density where both compressed and liquefied forms require more energy and suitable storage tanks which are heavy and difficult to integrate with the aircraft structure. This limits the use of hydrogen to flights that are less than 3,400 km.11 The first hydrogen aircraft is expected to enter the market in the 2030s and scale up through to 2050.
Operational & technical efficiencies
Increasing operational and technical efficiency is important in aviation’s decarbonisation pathway. Fuel consumption per passenger-kilometer has decreased 39% over the past 15 years at Compound Annual Growth Rate of 3.4%.12 Seat density and load factor optimisation have contributed 49% of the reduction but are approaching their natural limit to deliver further improvements. Fleet renewal accounts for 43% of the reduction, and new developments in aircraft design are a sustainable pathway for further fuel efficiency, as the last-generation aircraft is 20-25% less carbon intensive than the previous generation due to advanced materials, fuel-efficient engines, and improved aerodynamics.13 Operational efficiency can be further optimised through decreasing aircraft fuel burn through single-engine taxiing and smart air traffic management. These improvements represent the most immediately available and cost-effective measures that airlines can implement while more transformative technologies are being developed.
Government policy environment for aviation industry
Governments frameworks are pivotal in overcoming aviation’s decarbonisation barriers. In 2021, both European and American aviation sectors committed to net-zero carbon emissions by 2050. Among all effective decarbonisation strategies, more than half of decarbonisation of the aviation sector will depend on SAFs. However, the current challenge of widely adopting SAF is its production capacity and price. SAFs are much more expensive with their prices ranging from 2 to 9 times the price of traditional jet fuel, mainly due to the scale of the production. Therefore, governments play an important role in incentivizing and accelerating decarbonisation activities.
The UK’s Jet Zero strategy commits to invest in commercial-scale SAF plants through £165 million grant funding from the Advanced Fuels Fund to support the mandate of 2% SAF to be blended into conventional aviation fuels starting in 2025 and increasing linearly to 10% in 2030.14 The government also co-invests with industry in hydrogen and all-electric aircraft technologies through the Aerospace Technology Institute Programme to unlock innovative aerospace technology capability and support green jobs across the UK.
In comparison, the EU sets mandates under ReFuelEU without a comprehensive policy framework to incentivise cheaper production and more flexible rules of supply. To deliver the European Green Deal, the refuelEU regulation mandates aviation fuel suppliers a minimum share of SAF of 2% starting in 2025 and up to 70% by 2050.15 Without strong incentives in driving production, the EU is at risk of importing SAF to meet its targets which ultimately increases the cost of mobility throughout the EU. Countries like Spain and France plan or have already banned short-haul flights under 2.5 hours, where a train option exist to further eliminate emissions. In addition, the EU ETS reduces the free allowance that airline receives, 25% fewer in 2024 and 50% fewer in 2025.
The US Treasury released guidance on section 45Z Clean Fuel Production Tax Credit of offering up to $1.75 per gallon credit to producers for eligible SAFs that has a minimum reduction of 50% in lifecycle greenhouse gas emissions under the two CORSIA models. However, President Trump issued an Executive Order titled “Regulatory Freeze Pending Review” leaving the long-term impact of the 45Z guidance unclear.16
Sources
1 Airports Council International World.
2 IEA.
3 “Global aviation outlook: Air fares climb higher amid the unprecedented recovery of travel.” ING Think.
4 “Vision 2050 – Aligning Aviation with the Paris Agreement.” ICCT. June 2022. Pg. iii.
5 Jeffrey, Rebecca. “Cost is the biggest hurdle for hydrogen and electric aircraft.” Aircargo News.
6 The SAF Mandate: an essential guide. UK Department for Transport.
7 Alternative Fuels Data Center. U.S. Department of Energy.
8 “Beginner Guide to SAF.” Air Transport Action Group. Pg.7
9 Bushey, Claire. “US airlines ally with farmers to seek subsidies for corn as jet fuel.” Financial Times.
10 Lane, Jim. “The next big step for algae and sustainable aviation fuels.” The Digest.
11 “Zero-emission aviation: Opportunities on the horizon.” Climateworks Foundation.
12 “Fuel efficiency: Why airlines need to switch to more ambitious measures.” McKinsey & Company.
13 “Fleet renewal can help reduce aviation’s environmental impact as latest generation aircraft are quieter and more fuel efficient than their predecessors.” Airbus.
14 Sustainable Aviation Fuel (SAF) Mandate. UK government.
15 EUR-Lex. European Union.
16 WEF Global Aviation Sustainability Outlook 2025